Woven fiber reinforced plastics are receiving growing attention from the transportation industry because of their high performance to weight ratio. Due to its good geometric conformability, woven glass fiber reinforced plastics (GFRP) or carbon fiber reinforced plastics (CFRP) is most suitable for complex part geometries. The highly-automated composite thermoforming process of prepregs, as shown in Fig 1, was developed to reduce cycle time and manufacturing cost.
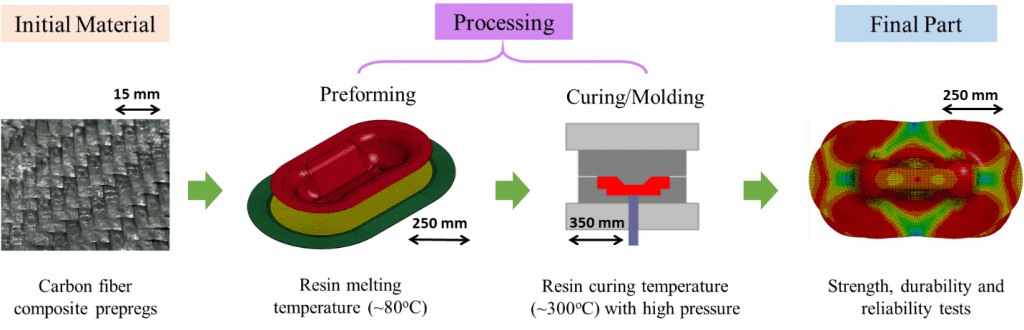
Fig. 1. Schematic of the carbon fiber composite thermoforming process
Material used in the first preforming step is the stacked flat layers of prepregs, which are woven CFRPs impregnated with uncured thermoset resin in desired fiber orientations. These layers are heated above the resin melting temperature to fully soften the prepreg and form into the part shape on a press. The formed part is then cured to harden the resin for the permanent shape.
Processing modeling of woven fabric composites Woven fabric composites usually undergo large in-plane shear deformation in the preforming process. The reorientation and redistribution of yarns after forming results in a significantly anisotropic material behavior. A non-orthogonal constitutive model to characterize this anisotropic behavior of woven composites has been developed. It enables us to accurately predict the yarn orientation and defects occurring in the preforming, such as wrinkles (Fig.2).

Fig. 2. Comparison between experiment and simulation with non-orthogonal constitutive model